Understanding Ancient Human Subsistence through the Application of Organic Residue Analysis on Prehistoric Pottery Vessels from the Korean Peninsula
Article information
Abstract
This study investigates prehistoric human subsistence and pottery use on the Korean peninsula through the organic residue analysis of archaeological potsherds. Ancient human subsistence is one of the core topics in Korean archaeology. However, due to the high acidity of sediments, which prevents the long-term preservation of organic remains, archaeologists have been short of critical information on how these early prehistoric dwellers lived. Ceramic vessels can contain well-preserved lipids originating from past culinary practices. For a better understanding of human subsistence on the prehistoric Korean peninsula, food-processing behaviors were reconstructed by analyzing ancient lipids extracted from a pottery matrix. The potsherd samples used in the analysis in this paper were collected from major prehistoric habitation sites. The results show that subsistence strategies differed according to both location and time period and reveal how organic residue analysis can contribute to a better understanding of prehistoric human subsistence strategies.
1. INTRODUCTION
Organic residue analysis has contributed to archaeology in various ways since it was first applied within the discipline. Following the discovery that lipids can be preserved in archaeological ceramics(Heron et al., 1991; Heron and Evershed, 1993; Evershed et al., 1994; Copley et al., 2005a; 2005b), the analysis of ancient lipids extracted from pottery matrices has become an important method of investigation that archaeologists use to gain a better understanding of ceramic artifacts’ function and local dietary patterns. While other approaches focus on reconstructing ancient vessel functions, such as use-wear analysis(Rice, 2005) and ethnographic studies(Rye, 1981; Costin, 2000; Rice, 2005), organic residue analysis investigates the items that were cooked or stored in a vessel by identifying the specific lipid compounds found therein(Craig et al., 2011; 2013). During the use of pottery vessels in prehistoric times(e.g., during culinary practices), fats, oils, and waxes originating from animals, insects, or plant products became entrapped within the vessels’ walls. These fats and waxes were largely protected from microbial and chemical degradation, as well as groundwater leaching, by the ceramic matrices(Figure 1). These organic residues can be extracted from potsherds and analyzed to infer what the vessels’ functions were hundreds and even thousands of years after the pottery was discarded by prehistoric people.
On the Korean peninsula, despite the presence of abundant archaeological material remains, information on most of the basic parameters involved in prehistoric subsistence is still lacking. Due to the high acidity of sediments, which does not allow the long-term preservation of organic matter, direct examination of the remains of resources is limited to shell middens and cave settings. Organic compounds have the advantage of being often preserved directly within archaeological ceramics(Copley et al., 2005a; 2005b; Charters et al., 2007), which is not the case with other methods of diet reconstruction, such as examination of faunal and floral remains.
This paper investigates organic residues extracted from pottery recovered from major archeological sites on the Korean peninsula(Figure 2)(Kwak and Marwick, 2015; Kwak, 2017; Kwak et al., 2017). To identify residues, gas chromatography-mass spectrometry(hereinafter GC-MS) and compound-specific isotope analysis(hereinafter CSIA) were used. GC-MS was used for the separation and identification of organic compounds in potsherds, and CSIA was employed for further isotopic analysis of specific compounds. Since fatty acids such as C16:0 and C18:0 are found in a range of different food products, CSIA can be used to further distinguish between their sources. CSIA uses gas chromatography-combustion-isotope ratio mass spectrometry(hereinafter GC-C-IRMS) to determine the stable carbon isotope(hereinafter δ13C) values of the principal fatty acids(C16:0 and C18:0), which are ubiquitous in archaeological ceramics. Many recent organic residue studies on potsherds have successfully detected the presence of different types of food groups, including animal fat, ruminant milk, marine resources(i.e., fish and mammal meat), freshwater resources, and C3, and C4 plants, through combining these two methods(Craig et al., 2011; 2013; Cramp et al., 2011). Within Korean archaeology, although several studies have analyzed ancient lipid residues with GC-MS(Lee et al., 1998; Ahn and Yu, 2002; Yun et al., 2007), the systematic application of CSIA with GC-C-IRMS on prehistoric pottery vessels has yet to be done. The purpose of this study is not to thoroughly trace prehistoric human subsistence practices in certain areas or sites on the Korean peninsula. Rather, the goal is to provide information about the general analytical procedure, the current findings, the advantages, and the limitations of this relatively new method to the wider communities of Korean archaeology studies and related disciplines.
2. MATERIALS AND METHODS
2.1. Organic residue analysis
Lipids, the organic solvent soluble components of living organisms, that is, the fats, waxes, and resins of the natural world, are the most frequently recovered compounds from archaeological contexts. Archaeologists have recovered a range of organic compounds from potsherds by using GC–MS. While organic compounds are relatively stable over a long period and are resistant to decomposition, they do degrade when exposed to oxygen and water. However, the ultimate purpose of organic residue analysis with GC-MS is to extract and identify the organic compounds that have been absorbed into the fabric of pottery. Studies have shown that these compounds are relatively well insulated and preserved within that fabric(Heron et al., 1991; Eerkens, 2001; 2005). Absorbed residues, unlike visible ones, cannot be removed from a potsherd by washing or scraping; they remain within the ceramic matrix until extracted by solvents(Reber and Evershed, 2004a). One of the most well-known approaches for interpreting archaeological organic residues using GC-MS relies on identifying a compound that is only found in a limited number of situations. Such “biomarkers” can help scientists to reconstruct the dietary life of prehistoric people(Heron and Evershed, 1993; Evershed, 2008a; 2008b). The biomarker approach seeks to identify a compound that is only found in a certain type of food group. These compounds, which vary for each case, constitute classes of biomarkers. These classes are present in different types of fats, for example, short-chain fatty acids in dairy fat, long-chain ω-(o-alkylphenyl) alkanoic acids in fish oil, unsaturated fatty acids in plant oil, cholesterol in animal fat, and plant sterols(e.g., b-sitosterol) in plant oil. However, they only occur in cases of good preservation of food residues. In many cases, these biomarkers can be masked by more abundant compounds, such as C16:0 and C18:0 fatty acids.
In most cases, a pot is reused multiple times and may be used to cook different kinds of food from one episode to another. Research with amino acids shows that the first use of a pot essentially saturates it with them, and seals it from further organic contributions. That is, amino acid residues trapped in a pot record only its first use(Fankhauser, 1997). On the other hand, fatty acids and other compounds tend to continuously accumulate in the fabric of a pot wall. Therefore, the result of the analysis is, in this case, more likely to reflect the entire usage of the pot. Generally, the result is assumed to represent the type of food group that was most frequently processed in it. However, this does not mean that the complication caused by its multiple usages can be disregarded. Besides, because of the different degradation rates and variable extraction rates of organic compounds, it is hard to tell exactly what types of food were processed in the pot only with GC-MS analysis. Reber and Evershed(2004b) pointed out that the complexity of the chemical interactions surrounding the preservation and degradation of absorbed organic residues should not be underestimated. To overcome this limitation and further clarify the origin of the organic compounds in a pot, a CSIA can be conducted. Conventional stable carbon isotope analysis has become a powerful method for tracing the dietary patterns of animals because their isotopic composition depends upon the food they eat(Bentley, 2007; Barton et al., 2009; Malainey, 2011). In archaeological settings, this method has been widely used on human remains for understanding subsistence patterns by separating them into C3 and C4 diets. With the introduction of GC-C-IRMS, the stable carbon isotope values of individual compounds in a mixture can now be measured with high precision(CSIA), providing a unique opportunity to conduct carbon isotopic analysis on the fatty acids that are insulated within the fabric of archaeological ceramics(Mottram et al., 1999). Scholars have successfully traced various types of food by measuring the δ13C values of the two most common fatty acids in archaeological pots, palmitic acid(C16:0) and stearic acid(C18:0), with CSIA using GC-C-IRMS(Reber and Evershed, 2004a; Craig et al., 2011; Cramp et al., 2011; Salque et al., 2013).
2.2. Analytical procedure
We extracted and analyzed lipids using the established protocol outlined by Correa-Ascencio and Evershed(2014). According to Correa-Ascencio and Evershed(2014), this new protocol achieves the extraction of lipid residues together with the simultaneous production of methyl esters of fatty acids. Approximately 5 g of each potsherd was sampled and the surface was cleaned using a drill(Dremel 3000, Dremel, USA) to remove any external contamination. The cleaned sample was ground to a fine powder in a glass mortar with a pestle and accurately weighed. The samples were methylated by adding 5 ml of H2SO4(sulfuric acid), MeOH(methanol), and heat(2% v/v, 70°C, 1 hour, vortex mixing every 5 minutes). The H2SO4-MeOH solutions containing the extract were transferred to test tubes and centrifuged for 10 minutes(2500 r/min). The clear solution was transferred to clean culture tubes and 2 ml of ultra-purified water was added. Then, 4 ml of hexane was added and vortex-mixed to recover the lipids(x2).
The extracted lipids, or fatty acid methyl esters(FAMEs) were analyzed using a 6890 N Network GC system with a 5979 mass selective detector from Agilent Technologies. The GC was equipped with a fused silica capillary column(J&W, 6890N/5979, Agilent Technologies, USA) (DB5-MS, J&W, USA, 60 m × 0.32 mm, 0.25 μm film thickness). The mass spectrometer was operated in the full-scan mode. Helium was the carrier gas and the GC oven was programmed as follows: a two-minute isothermal process at 50°C followed by an increase to 350°C at a rate of 10°C/min and, following this, the temperature was held at 350°C for 10 minutes. Peaks were identified based on their mass spectral characteristics and GC retention times and also by comparison with a NIST mass spectral library. Each sample was analyzed once. CSIA was performed using a thermo GC/C-IRMS system composed of a Trace GC Ultra gas chromatograph(Trace GC Ultra, Thermo Electron Corp., ITA) coupled to a Delta V Advantage Isotope Ratio Mass Spectrometer through a GC/C-III interface(Delta V, Thermo Electron Corp., DEU). Compound identification support for the CSIA laboratory was provided by a Varian CP3800 gas chromatograph coupled to a Saturn 2200 ion trap MS/MS (CP3800/Saturn 2200, Varian, Inc., USA). The FAMEs dissolved in hexane were injected in splitless mode and separated on a Varian Factor Four VF-5ms column(30 m × 0.25 mm ID, 0.25 μm film thickness). Once separated, the FAMEs were quantitatively converted to CO2 in an oxidation reactor at 950°C. Each sample was measured ten times. The precision of the measured δ13C values was determined and corrected using working standards composed of several FAMEs calibrated against NIST standard reference materials(Colonese et al., 2015).
2.3. Sampling
According to the experimental analysis of Evershed(2008a), the rim and upper body parts of pots are where the organic residues are the most concentrated after cooking(Figure 3A). Ethnographic observations have shown that high-temperature boiling is regarded as a particularly effective cooking method in the preparation of faunal and floral resources in pots(Stahl, 1989; Crown and Wills, 1995; Wandsnider, 1997). During this process, the convection currents of boiling water push extracted lipids from foodstuffs to the pot wall. Once lipids float on water, they tend to accumulate and penetrate the wall of the upper body and rim of the pot. The experimental GC-MS analysis on Iron Age pottery from Eupha-ri, Hoengseong county, Gangwon province(Figure 2), also confirms Evershed’s observation. Three potsherds were selected: one rim, one upper body, and one bottom from a single pot(Figure 3B). In the GC-MS results, each of the peaks in the chromatogram with different retention time(along the horizontal axis) indicates a different compound(Figure 4). These compounds might indicate faunal or floral remains absorbed in the pot matrix. The height and width of each peak determine its area, which indicates the amount of individual compound. Of the three potsherds, only the bottom one did not have enough concentration of a fatty acid that could be properly analyzed(Figure 4). The results of the experiments confirm that Evershed’s criteria can be applied to the context of the Korean peninsula. This also indicates that boiling food in a pot was regarded as an effective cooking method among the prehistoric dwellers of the Korean peninsula.
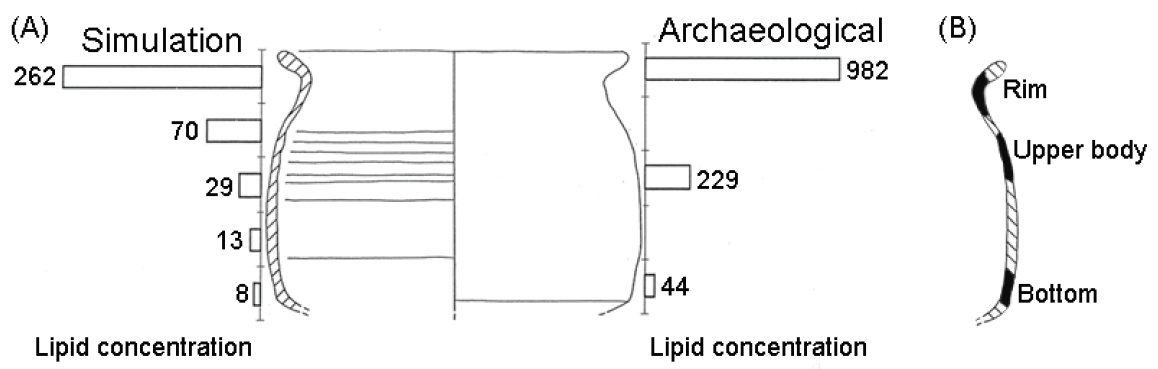
(A) The side profile of pot showing the lipid concentrations(µg lipid/g-1 sherd). Left: the result of simulation experiment. Right: the actual lipid concentration data from archaeological sherds(adapted from Figure 2 of Evershed, 2008a). (B) Schematic profile view that shows the approximate locations of sampled portions of the pot excavated from Eupha-ri for the experimental analysis.

The results of experimental GC-MS analysis on the potsherds from Eupha-ri site. The X-axis indicates retention time, Y-axis indicates relative abundance. The height and width of peak determines the area of peak, which indicates the amount of individual compound. (A) rim, (B) upper body, and (C) bottom. The bottom part shows significantly low concentration, when compared with rim or upper body part.
3. TRACING SUBSISTENCE BEHAVIORS IN THE PREHISTORIC KOREAN PENINSULA USING ORGANIC RESIDUE ANALYSIS
Below, some of the findings on prehistoric human subsistence on the Korean peninsula are presented. Specifically, there is a focus on the subsistence living of the Neolithic and Bronze Ages. However, to meet the purpose of this paper, specific cases are not thoroughly addressed.
3.1. Learning from GC-MS results
As mentioned above, in the GC-MS results, each of the peaks on the chromatogram with a different retention time indicates a different compound. Generally, the most frequently observed compounds in archaeological lipid residues are palmitic(C16:0) and stearic(C18:0) fatty acids(Evershed, 2008b). Some compounds observed on the chromatogram only exist in certain types of foods. Accordingly, they are known as biomarkers and they can help to narrow down the identity of items processed in a pot. Examples of these biomarkers are short-chain fatty acids in dairy fats, unsaturated fatty acids in plant oils, cholesterol in animal fats, and plant sterols in plant oil. Also, distributions of triacylglycerols in ancient fats from pots can provide reasonable evidence of the presence of animal fats and dairy products(Mukherjee, 2004). In addition, phytanic acid, pristanic acid, and 4, 8, 12-TMTD are isoprenoid compounds that are mostly found in particularly high concentrations in marine animals(Hansel et al., 2004; Solazzo and Erhardt, 2007; Evershed et al., 2008; Craig et al., 2011; Vetter and Schröder, 2011). Together with thermally produced, long-chain ω-(o-alkylphenyl) alkanoic acids, these compounds are indicators of aquatic/marine resources(Hansel et al., 2004; Evershed et al., 2008a; Craig et al., 2011). Generally, marine commodities contain high concentrations of polyunsaturated fatty acids. However, these unsaturated fatty acids hardly survive in archaeological pottery, as they are particularly susceptible to postdepositional processes(Evershed et al., 2008a). During the heating of polyunsaturated fatty acids, ω-(o-alkylphenyl) alkanoic acids, which are stable compounds, can be formed(Hansel et al., 2004). Since all cooking processes include heating, this offers a means of detecting foodstuffs that contain polyunsaturated fatty acids, such as marine resources, from archaeological pottery vessels.
Figure 5 shows the results of the GC-MS analysis of the two samples from Sosa-dong(SOS049) and Beombang(BB026). The organic compounds of most samples from the Korean peninsula analyzed so far have been dominated by palmitic(C16:0) and stearic(C18:0) fatty acids(Kwak and Marwick, 2015; Kwak, 2017; Kwak et al., 2017). This means that most of the organic compounds relating to the original contents of the prehistoric vessels have been highly degraded during several thousand years of post-depositional processes(Evershed, 2008b). Still, what can be understood from high concentrations of stearic(C18:0) acids is that they may indicate an animal source(Enser, 1991; Copley et al., 2005c; Evershed et al., 2002). Besides this, with the results of the GC-MS analysis, both major short- and long-chain saturated fatty acids could be identified, comprising C13:0, C14:0, C15:0, C17:0, C20:0, C22:0, C23:0, and C24:0. In addition, some of the samples from Gosan-ri(Neolithic), Dongsamdong(Neolithic), Beombang(Neolithic), Sosa-dong (Bronze Age), Kimpo-Yangchon(Bronze Age), and Songguk-ri(Bronze Age) showed the presence of phytanic acid, indicating the possibility that those pots were used for processing aquatic resources(Figure 5)(Kwak and Marwick, 2015; Kwak et al., 2017). Although the amount of phytanic acid is quite small when compared with major saturated fatty acids such as palmitic and stearic acids, this is normal in degraded archaeological residues(Craig et al., 2011). Based on the local geography of these sites, it is quite probable that these Neolithic and Bronze Age dwellers procured aquatic resources (Figure 2). For example, the Kimpo-Yangchon site is only four kilometers from the Han River and the Sosa-dong site is only about 2.5 kilometers north of the Anseong Stream. Both sites are also quite close to the coastline of Korea’s West Sea. Furthermore, during the excavation of the Sosa-dong site, a total of 17 net-sinkers were found(Korea Institute of Heritage, 2008). However, phytanic acid can also be traced in the tissues of ruminants and other terrestrial animals. Therefore, it should be noted that phytanic acid alone is not a reliable indicator of an aquatic/marine diet without other biomarkers(Heron and Craig, 2015). Since we were not able to detect other aquatic biomarker such as ω-(o-alkylphenyl) alkanoic acids, only with the GC-MS results, we cannot argue that our samples were used to process/cook marine resources.

The results of GC-MS analysis on the potsherds from (A) Bronze Age Sosa-dong(sample code SOS049) and (B) Neolithic Beombang site(sample code BB026). The X-axis indicates retention time, Y-axis indicates relative abundance. 5-α Cholestane was added as an internal standard for quantification(IS: 100 ng/µL).
3.2. Isotopic implication: CSIA
Different types of foodstuffs(e.g., beef, pork, fish) tend to have different ranges of δ13C values of C16:0 and C18:0 fatty acids. Although these values were obtained from modern fauna and flora, they have been reliably employed as references for many archaeological studies(Reber and Evershed, 2004a; Craig et al., 2011; 2013; Cramp et al., 2011). Ideally, the reference database for CSIA should be based on the modern samples of fauna and flora from the same region where the archeological materials are collected. However, modern commercial farming with supplements makes it difficult to directly compare δ13C values from archeological materials with those from modern samples. To overcome this issue, samples for reference databases have been collected from wild fauna and flora(Craig et al., 2011; 2013). Unfortunately, in the case of the Korean peninsula, wild terrestrial mammals are extremely rare. In this setting, one method is to employ the reference database of Craig et al.(2013), where the reference data were generated using modern wild fauna in Japan. However, one might question whether the δ13C values of the modern samples from the Japanese archipelago are comparable with the archaeological ones from the Korean peninsula despite the geographical proximity.
To overcome this inherent limitation, the approach of Salque et al.(2013) was adopted for the interpretation of the results. This approach focuses on the change in δ13C values during the metabolic and biosynthetic processes of animal sources and allows for the complete removal of the exogenous factors related to the local environment. It uses the Δ13C(δ13C18:0 - δ13C16:0) proxy, which can separate fats based on the physiological differences between the animals regardless of the differences of their diets or the surrounding ecosystem(Evershed, 2008b). With this approach, a distinction between non-ruminant adipose, ruminant adipose, and ruminant milk can be made.
Figure 6 and Table 1 show the results of the CSIA of the palmitic(C16:0) and stearic(C18:0) fatty acids extracted from the potsherds excavated from the Neolithic Beombang shell midden site and the Bronze Age Songguk-ri site(Kwak et al., 2017). At Songguk-ri, the samples were collected from the in situ floor of semi-subterranean pit-houses and pit features, as these potsherds are regarded as the most likely to be related to culinary practices. A total of 27 samples were collected from 16 pit-houses and 2 pit features. Among them, 18 samples(67%) contained lipid residues. In the case of Beombang, 8 out of 32 samples(25%) contained lipids. The results of the isotope analysis show some interesting characteristics of these prehistoric dwellers’ diet. Within Korean archaeology, the traditional perception of Neolithic subsistence living is that it was a combination of hunting, fishing, and gathering(Kim, 2003). In particular, coastal hunter-gatherers are regarded as having intensively procured marine resources and created hundreds of shell middens throughout the peninsula. The initial domestication of the millet family started as early as in the middle phase of the Korean Neolithic(ca. 5500 BP), but is assumed to have remained at a low level. From the Bronze Age, with evidence of full dress farming, including dry fields, irrigated paddies, harvesting tools, and paleobotanical data, it is assumed that harvested crops such as rice and barley formed a significant portion of the diet of these ancient people(Ahn, 2000). The isotopic results on Beombang and Songguk-ri reflect these trends. Beombang is one of the well-known coastal shell midden sites in the southern part of the Korean peninsula. Songguk-ri, probably the most significant and well preserved Middle to Late Bronze Age village site on the entire peninsula, is known for representing the pinnacle of an agricultural economy based on intensive rice farming. Most of the samples from Beombang show relatively high δ13C values, indicating that the pots were mainly used for processing marine resources/C4 plants(e.g., millet)(Figure 6) (Evershed et al., 2008b; Dunne et al., 2012; Salque et al., 2013; Kwak et al., in press). On the other hand, the δ13C values of the Songguk-ri samples were quite low in general, indicating that the dwellers mostly relied on terrestrial resource/C3 plants(e.g., rice)(Evershed et al., 2008b; Dunne et al., 2012; Salque et al., 2013; Kwak et al., 2017). However, at the same time, the CSIA results also reveal certain subsistence patterns of these Bronze Age “farmers” that have been somewhat overlooked so far. By employing the approach of Salque et al.(2013), evidence of ruminant hunting at Songguk-ri was found, as about half of the samples analyzed were identified as ruminant adipose fat(Figure 6)(Kwak et al., 2017). Within Korean archaeology, the role of subsistence strategies besides farming in Bronze Age people’s diets, for example, hunting terrestrial animals, has largely been underestimated because of the poor preservation of organic remains. Our study offers an approach to investigating the relative contribution of various natural resources to the prehistoric diet, which overcomes this problem of differential preservation in archaeological deposits. In the case of Songguk-ri, the results suggest that these farmers relied on hunting wild animals to a greater degree than expected. It is worth noting that the CSIA result from the Sosa-dong Early Bronze Age site was slightly different from that of Songguk-ri(Figure 7, Table 2). As at Songguk-ri, the Sosa-dong samples were collected from the in situ floor of semi-subterranean pit-houses. A total of 37 samples were collected from 18 pit-houses. Among them, 27 samples(73%) contained lipid residues. Compared to the subsistence practices of Songguk-ri, it shows relatively wider resource procurement(Kwak, 2017). A similar pattern was witnessed at the Neolithic Gosan-ri site of Jeju Island. The result provides a rare chance to investigate the subsistence pattern of early Holocene maritime hunter-gatherers. When compared with the result from Beombang(Figure 6), Gosan-ri shows a much wider range of resource utilization despite the similarity in the geographical setting(Kwak, 2018). This indicates that even in a similar geographical setting, the subsistence patterns of hunter-gatherers and prehistoric farmers can vary significantly depending on the environmental situation that these people faced.
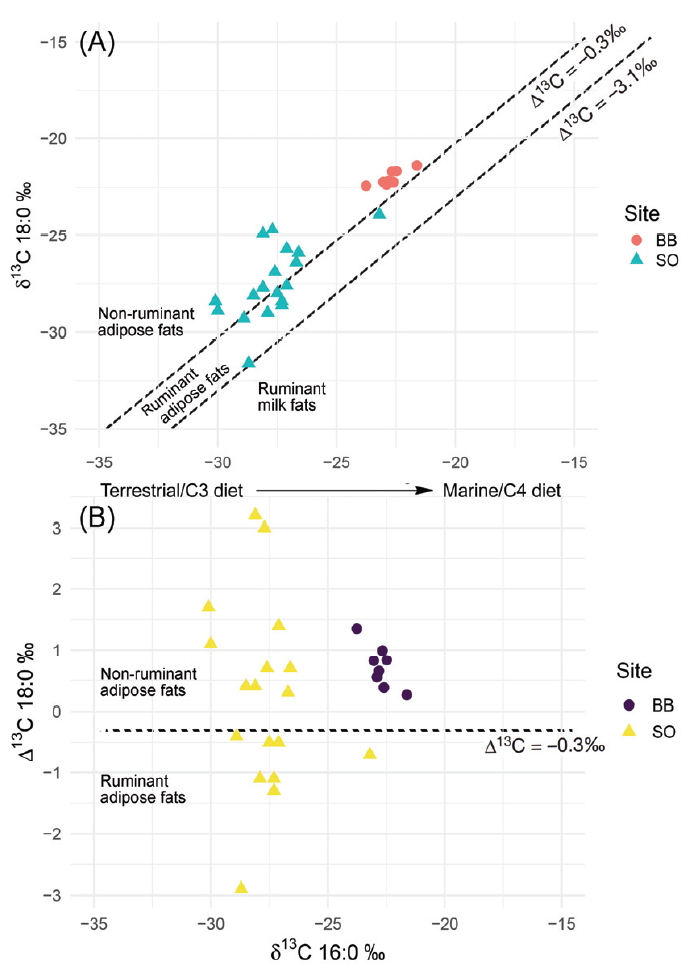
CSIA results of potsherd samples from Neolithic Beombang(BB) and Bronze Age Songguk-ri(SO) sites. δ13C18:0 = δ13C value of 18:0 fatty acid. δ13C16:0 = δ13C value of 16:0 fatty acid. Δ13C 18:0 = δ13C18:0 - δ13C16:0. (A) Most of BB samples show relatively high δ13C values. Low δ13C values of SO samples indicate contribution of C3/terrestrial resource. (B) About half of the SO samples were identified as ruminant adipose fat.

CSIA results of potsherd samples from the Early Bronze Age Sosa-dong(SS) site. δ13C18:0 = δ13C value of 18:0 fatty acid. δ13C16:0 = δ13C value of 16:0 fatty acid.
4. CONCLUSION
This research aims to inform general analytical procedure, the current findings, the advantages, and the limitations of organic residue analysis for communities of Korean archaeology studies and related disciplines. Most of the findings reveal that organic residue analysis might seriously contribute to a better understanding of prehistoric human subsistence strategies. The relatively high acidity of the sediments, which does not allow for the long-term preservation of organic remains, is the toughest hindrance to subsistence studies within Korean archaeology. Since organic residue analyses deal with absorbed residues in pottery matrices, past subsistence behaviors even in the absence of faunal or floral remains can be understood. For example, at Songguk-ri, the results suggest that these farmers relied on hunting wild animals to a greater degree than expected. It should be noted that the study does have limitations. This approach may not reflect the entire subsistence of sites analyzed for several reasons. These include not sampling every habitation location at these sites, boiling foods in a pot is not the only method for preparing food for consumption, and there may be other methods of food preparation that did not involve pottery. The residue analysis may only capture a narrow singular aspect of food preparation and consumption. There is also the possibility that the pots may have been used to store items unrelated to human subsistence, and that not all of the compounds identified are relevant to dietary behaviors. Moreover, the result of the CSIA is assumed to represent the type of food groups that were most often processed. This means that even though the δ13C values of a certain potsherd indicate the presence of ruminant fat, it was possibly also used to a lesser degree for cooking other foods, such as rice. These limitations constrain the generality of the findings. Nevertheless, it is expected that this approach can be applied to other prehistoric and historic habitation sites on the Korean peninsula and other locations with similarly poor organic preservation to further understanding of human subsistence practices and pottery use.
Acknowledgements
This work was supported by the Ministry of Education of the Republic of Korea and the National Research Foundation of Korea(NRF-2017S1A5B5A07062030). A special thanks to Dr. Richard Evershed, Dr. Julie Dunne, and Dr. Marisol Correa-Ascencio for sharing their knowledge related to the GC-MS and CSIA.